KEYWORDS
Microalgae
Sustainability
Cultivation
Astaxanthin
Environment
Although microalgae certainly represent a novel, promising source of several bioactive compounds, less attention has been paid to the difference between potential cultivation techniques and their associated environmental and sustainability footprint. Here, location, water availability and quality, cultivation technique, energy source, energy requirements, volumetric productivity, downstream approach and carbon footprint are key factors in the overall sustainability profile of a given approach. The paper will compare different approaches to microalgae cultivation with regards to sustainability impact and other environmental considerations.
Abstract
The application field for microalgae derived ingredients has been growing at a rapid pace over the last decade. In addition to biomass-based products and ingredients such as Spirulina and Chlorella there is also an increasing market for well-known compounds such as omega 3‘s (DHA and/or EPA) and beta glucan and high-value specialty ingredients such as astaxanthin, fucoxanthin, phycoerythrin and phycocyanin. In many ways though the microalgae industry is still in its infancy and quality and product related standards are being implemented and agreed upon.
As the microalgae industry is expanding, value-chain stakeholders and end-consumers are also shifting more focus towards sustainability related aspects of the process. This can refer to everything from raw ingredient sourcing, energy and water inputs, cultivation approach and overall environmental/carbon footprint of both the production company as such and the finished end-product.
When looking at each specific category separately it is evident that a systematic and quantifiable approach needs to be taken when comparing different production methods of microalgae-derived high-value ingredients. Established methods such as Life-cycle-assessment are certainly a good starting point but in addition, certain algae-specific metrics need to be incorporated. Below is a brief summary of some of the different categories in question. The discussion in only meant to be a short outline of relevant parameters to consider rather than an extensive in-depth-analysis.
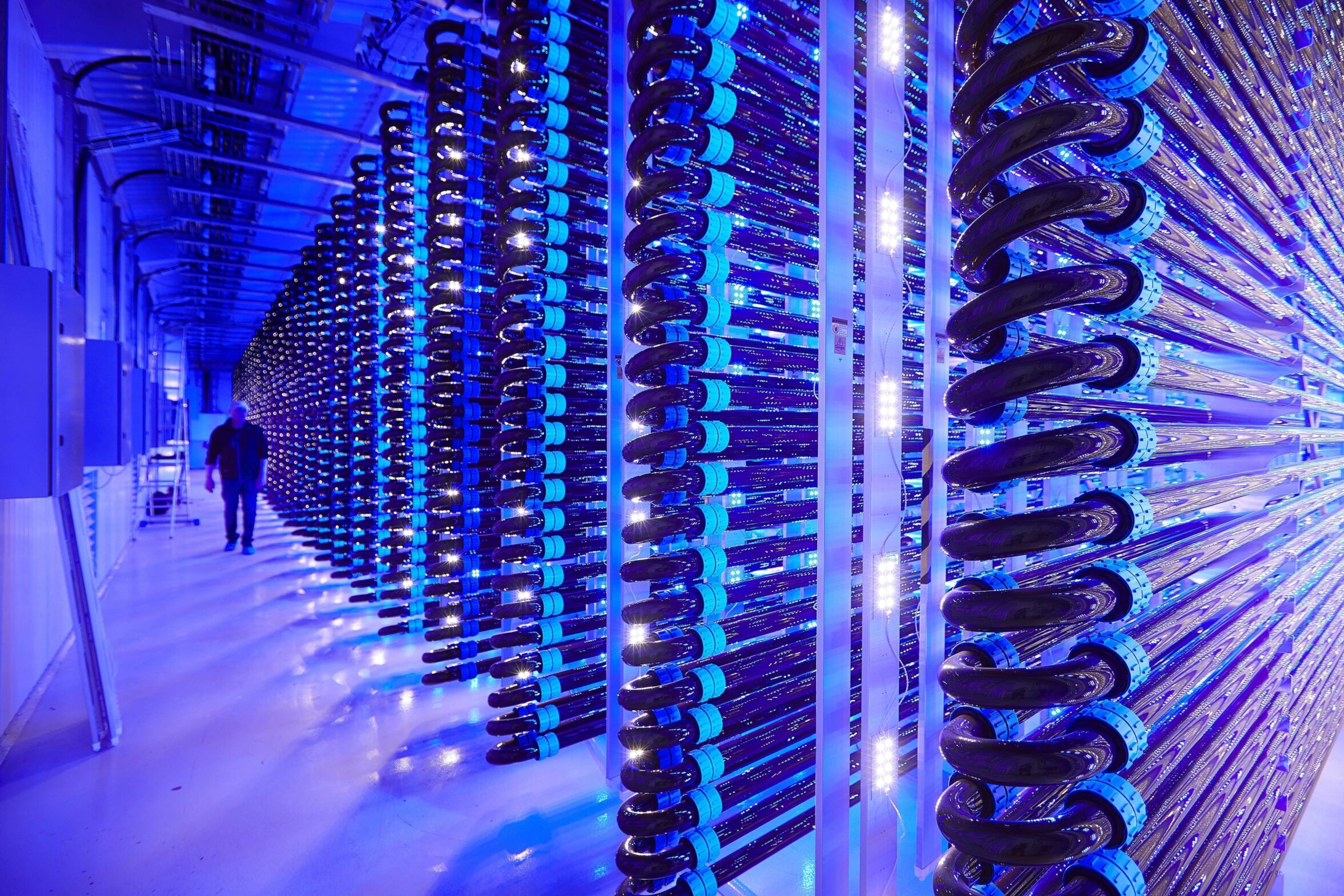
Location
Location in this context can refer to both geographical location but also the distinction between cultivation that takes place outdoors and cultivation that takes place indoors. In both cases though, access to a sustainable, stable, and renewable energy source is of critical importance.
Outdoor operations are usually located in warm, sunny climates and have the general sustainability advantage of using natural sunlight to drive the photosynthesis and thereby requiring less energy overall. The disadvantages include increased contamination risk and bioburden, low productivity, limited light utilization and a stronger need for external temperature adjust which can increase the environmental footprint substantially.
Indoor operations are usually more energy intensive as the process is often driven by artificial high-energy lighting. The advantages however include full parameter control (temperature, pH etc.), enhanced productivity (partly due to being able to cultivate both day and night), lower contamination risk and lower energy requirements for temperature adjustment. Locations with stable, low-temperature climates, low bioburden and access to renewable energy are therefore best suited for indoor microalgae cultivation.
Water availability & quality
Water is the single most important ingredient in a microalgae cultivation. Microalgae cultures are predominantly made up of water (biomass density rarely exceeds 10 g/L and is often around 1-4 g/L) so it goes without saying that water availability and quality are critical factors. Sustainability and environmental considerations are tightly linked to the water source in many ways:
- Sustainable, steady water access is of primary importance and the water use must not be at the expense of other industries or general use
- Chemical pre-treatment of the water is needed in locations with poor water quality, and this has a negative impact on the overall sustainability and environmental profile
- The suitability of the local water profile needs to be aligned with the intended culture medium to be used and the microalgae species in question. If the profile is not suitable, adjustments need to be made and these can have negative environmental impacts
- Controlling water use by ensuring that evaporation rates are minimized is a sustainable step to take. Evaporation rates in open pond, outdoor systems can exceed 20% per day but in closed, indoor systems the evaporation rates can be maintained well below 1%
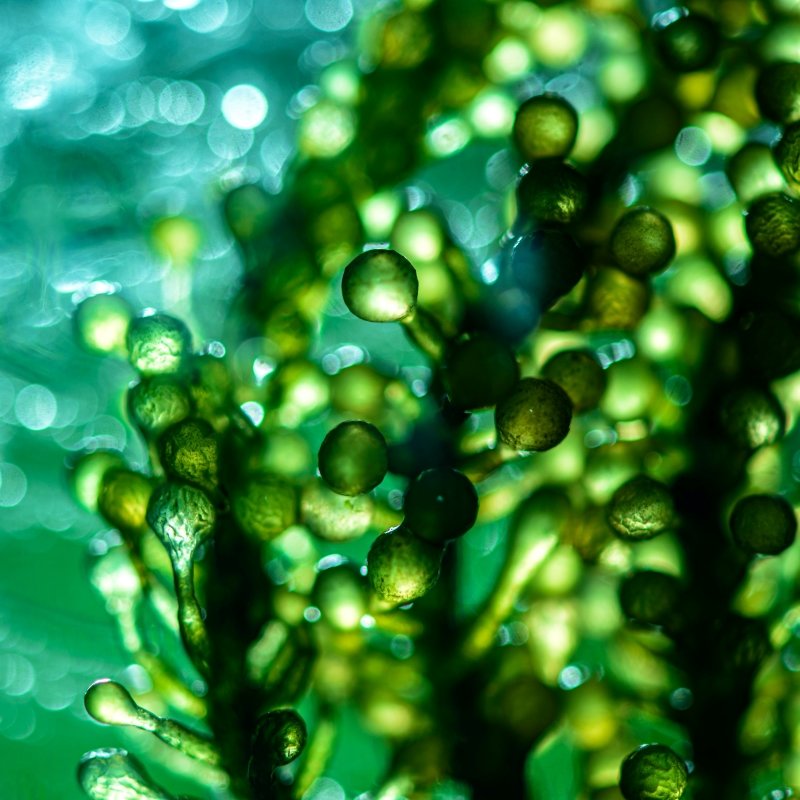
Production technique
Even though microalgae are predominantly cultivated using a phototrophic approach and therefor consume carbon dioxide and convert it to biomass and oxygen, the “environmental service” associated with this process is not as obvious as it might appear since the source of the carbon dioxide itself also plays a role. This is most evident when comparing the contrasting scenarios of using carbon dioxide that has been removed directly from the atmosphere and concentrated (net removal of carbon dioxide) or when using carbon dioxide commercially produced in a conventional chemical process (thereby increasing the overall carbon dioxide footprint). In addition, some cultivation approaches (e.g., mixotrophic cultivation) use other carbon sources such as simple sugars and this can negatively impact the overall environmental footprint as the carbon source might not be locally available and therefor be subject to transportation etc.
Certain raw materials (macro- and microelements) are needed to provide optimum nutrients in the microalgae cultivation process. The sustainability profile of these compounds is very different based on the source and the location. One example is the nitrogen source as this can be anything from chemically synthesized nitrate to a natural, organic source such as “Chilean nitrate” to a biorefinery source where nutrient-rich waste streams are utilized as fertilizer for microalgae cultivation.
As previously mentioned, volumetric productivity can vary significantly between indoor and outdoor systems, even when comparing the same microalgae strains. High productivity is positively correlated with sustainable resource use as less water and land is needed to produce each kilogram of biomass. High productivity systems also tend to require less cleaning due to lower contamination load and this reduces the need for using harsh chemicals that have a negative impact on the overall environmental profile.
Dewatering is usually the first step in any microalgae downstream process and there are several different ways to perform this e.g., filtration, flocculation and centrifugation. These approaches differ somewhat in energy requirements and therefor environmental impact. In many cases, dewatering is followed by a drying step which can also differ in terms of kilowatts needed to dry each kilogram of biomass. Finally, many applications require further processing such as cell cracking an extraction/separation. In general, environmentally friendly methods such as supercritical carbon dioxide extraction (where most of the carbon dioxide is recycled) is preferred over chemical/solvent extraction using harmful chemicals.
Other sustainability considerations
In addition to the abovementioned factors and their associated environmental footprint, other, more common things also have to be taken into consideration. These are factors such as transportation of goods, waste management procedures, whether vehicles are powered by electricity or fossil fuels and numerous other environmentally related aspects.
Overall, this discussion demonstrates that although microalgae cultivation for the production of bioactive compounds is an inherently environmentally friendly process, many details have to be taken into consideration to assess the overall sustainability profile and to compare products produces by different cultivation techniques and in different locations.
References and notes
- Still feeling good: The US wellness market continues to book, McKinsey (2022) available at https://www.mckinsey.com/industries/consumer-packaged-goods/our-insights/still-feeling-good-the-us-wellness-market-continues-to-boom
- Personalized Nutrition Market to Perceive Promising Growth of USD 20.14 Billion with CAGR of 10.50% By 2029: Size, Share, Industry Analysis, Trends And Value Forecast, Data bridge market research (2022) available at https://www.globenewswire.com/news-release/2022/09/16/2517398/0/en/Personalized-Nutrition-Market-to-Perceive-Promising-Growth-of-USD-20-14-Billion-with-CAGR-of-10-50-By-2029-Size-Share-Industry-Analysis-Trends-And-Value-Forecast.html
- Hot or not: Where European VC funding went in 2022 available online at https://pitchbook.com/news/articles/2022-europe-israel-vc-funding-breakdown
- Nutrition Business Journal Personalized nutrition special report (2022) available at https://store.newhope.com/collections/reports/products/personalized-nutrition-special-report-2022
- Europe Sport nutrition market 2023-2028 available online at https://www.mordorintelligence.com/industry-reports/europe-sports-nutrition-market
- Global metabolic testing market by 2030 - https://www.globenewswire.com/en/news-release/2022/10/28/2543696/0/en/Metabolic-Testing-Market-Worth-US-1125-26-million-by-2030-Growth-Plus-Reports.html
- Berry, S.E et al .,Human postprandial responses to food and potential for precision nutrition. Nat Med26, 964–973 (2020). https://doi.org/10.1038/s41591-020-0934-0
- Zeevi et al. Personalized nutrition by prediction of glycemic responses. Cell Vol:163, issue nr.5: 1079-1094, Nov 19 (2015) doi https://doi.org/10.1016/j.cell.2015.11.001
- Inez Trouwborst, et al.Cardiometabolic health improvements upon dietary intervention are driven by tissue-specific insulin resistance phenotype: A precision nutrition trial, Cell Metabolism, Volume 35, Issue 1, 2023, Pages 71-83.e5, ISSN 1550-4131, https://doi.org/10.1016/j.cmet.2022.12.002
- Radtke, M.D.et al. Validation of Diet ID™ in Predicting Nutrient Intake Compared to Dietary Recalls, Skin Carotenoid Scores, and Plasma Carotenoids in University Students. Nutrients2023, 15, 409. https://doi.org/10.3390/nu15020409
- Moyen A et al. Relative Validation of an Artificial Intelligence–Enhanced, Image-Assisted Mobile App for Dietary Assessment in Adults: Randomized Crossover Study J Med Internet Res 2022;24(11):e40449 doi: 10.2196/40449
- Barron, E, Bradley, D, Safazadeh, S, et al. Effectiveness of digital and remote provision of the Healthier You: NHS Diabetes Prevention Programme during the COVID-19 pandemic. Diabet Med. 2023; 00:e15028. doi:10.1111/dme.15028
- Ethical guidelines for trustworthy AI (2019) available at https://digital-strategy.ec.europa.eu/en/library/ethics-guidelines-trustworthy-ai
- European commission. Regulation of the European Parliament and of the council laying down harmonised rules on artificial intelligence (artificial intelligence act) and amending certain union legislative acts (2021) https://artificialintelligenceact.eu/the-act/
- Qina LDA. The Hub for data and insights in Personalised nutrition. Available online at https://qina.tech